Welcome to The Century of Biology! This newsletter explores data, companies, and ideas from the frontier of biology. You can subscribe for free to have the next post delivered to your inbox:
and I explored biotech platform strategy. Today, let’s zoom farther out to explore ideas for biotech-enabled space exploration. Stay tuned for more detailed company analysis next time.Enjoy! 🧬
Rocket science is hard. Designing systems capable of launching materials—including humans—into orbit and then smoothly landing back on terrestrial ground is one of the greatest feats of engineering we’ve achieved. But surviving in space will be even harder.
In his brilliant 2020 keynote on the future of genetic engineering, Asimov CEO Alec Nielsen made the following argument:
And space, the final frontier. Everyone talks about rocket science being the hard problem about outer space. But biology is the really hard thing about outer space. We need to able to manufacture food and therapeutics in the defined closed system of a space station. One day, we need to be able to terraform entire planets to be hospitable for life. We need to be able to engineer biomes. These are biology problems, not rocket problems.
Aerospace engineers have been hard at work. The cost of launching things into space has plummeted in recent decades, and soon, it’s going to get even cheaper. We’ve also accomplished stunning acts of outer space coordination, like the tennis court-sized Webb telescope that unfurled itself from its original “origami-style” configuration while in orbit.
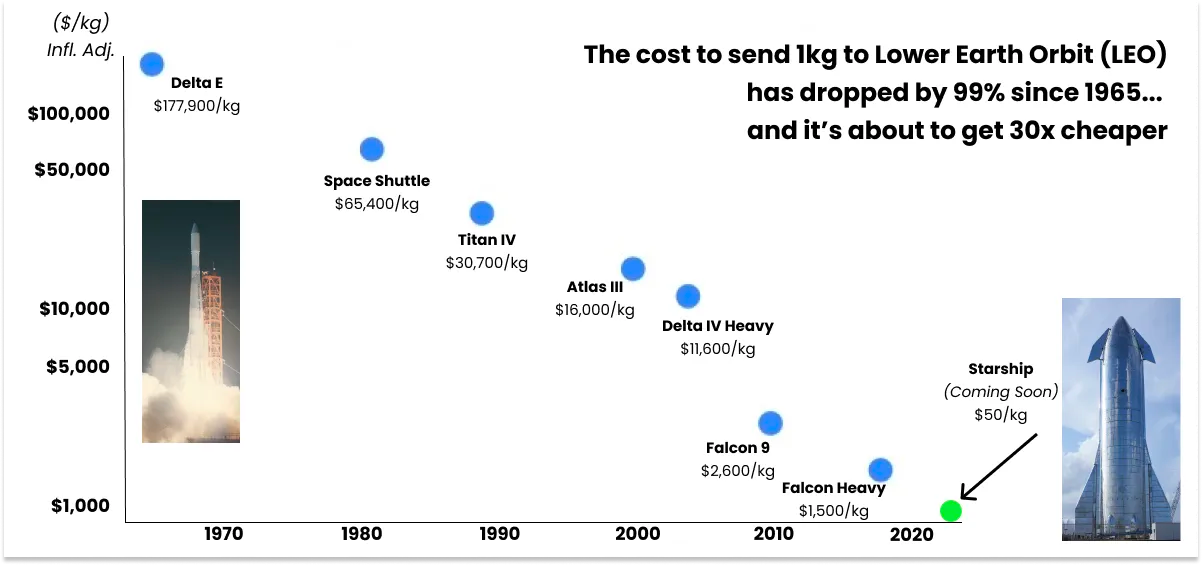
As rockets get cheaper and bigger, the bottleneck for space colonization will be our ability to support biological life outside of Earth’s atmosphere.
Every terrestrial organism is hyper-adapted to survive in Earth’s specific environmental conditions, much like a machine learning model that is drastically overfit to its training data. Never once has there been a selective pressure to optimize for anywhere else. Developing sufficient infrastructure to support life in space will be an extraordinary challenge for biotech.
Why do this in the first place? If we’re so exquisitely evolved for Earth, why try to leave? Beyond the philosophical and aesthetic view that life should expand outwards into the stars—which I firmly believe—there are more pragmatic reasons for developing better outer space survival tools.
Space technology drives terrestrial technology forward. The history of NASA spinoff technologies is so immense that it has its own publication. We’re talking about the development of LASIK, cochlear implants, improved fire-fighting equipment, CMOS image sensors, GPS error correction, better simulation software, solar cells, and much, much more.
Space survival is the ultimate pressure test for producing miniaturized, modular, failure-proof biotechnologies. These same tools could enable the full biologization of the economy, ensuring sustainable abundance for billions of humans on Earth.
So today, let’s turn our attention outwards. We’re going to explore the landscape of ideas for biotech-enabled space exploration. In doing so, we’ll see that biotech is about a lot more than just making drugs.
Microbial machinery for space habitats
Humans need a lot of things to survive. We need reliable sources of water, breathable air, medicines, and materials for constructing tools and habitation. Earth is rich with these resources, but space isn’t. The farther outward we venture, solutions for managing biological waste, recycling, manufacturing, and local resource utilization will also become increasingly necessary.
Earlier this year, leading space biotechnologists published a sweeping review outlining how microorganisms may provide solutions to each of these problems.
Let’s start with the basics: a need for breathable air. Our oxygenated atmosphere on Earth is the byproduct of billions of years of photosynthesis by cyanobacteria and plants. Light energy and CO2 go in, chemical energy and oxygen come out. Biology is capable of air remediation at the planetary scale.
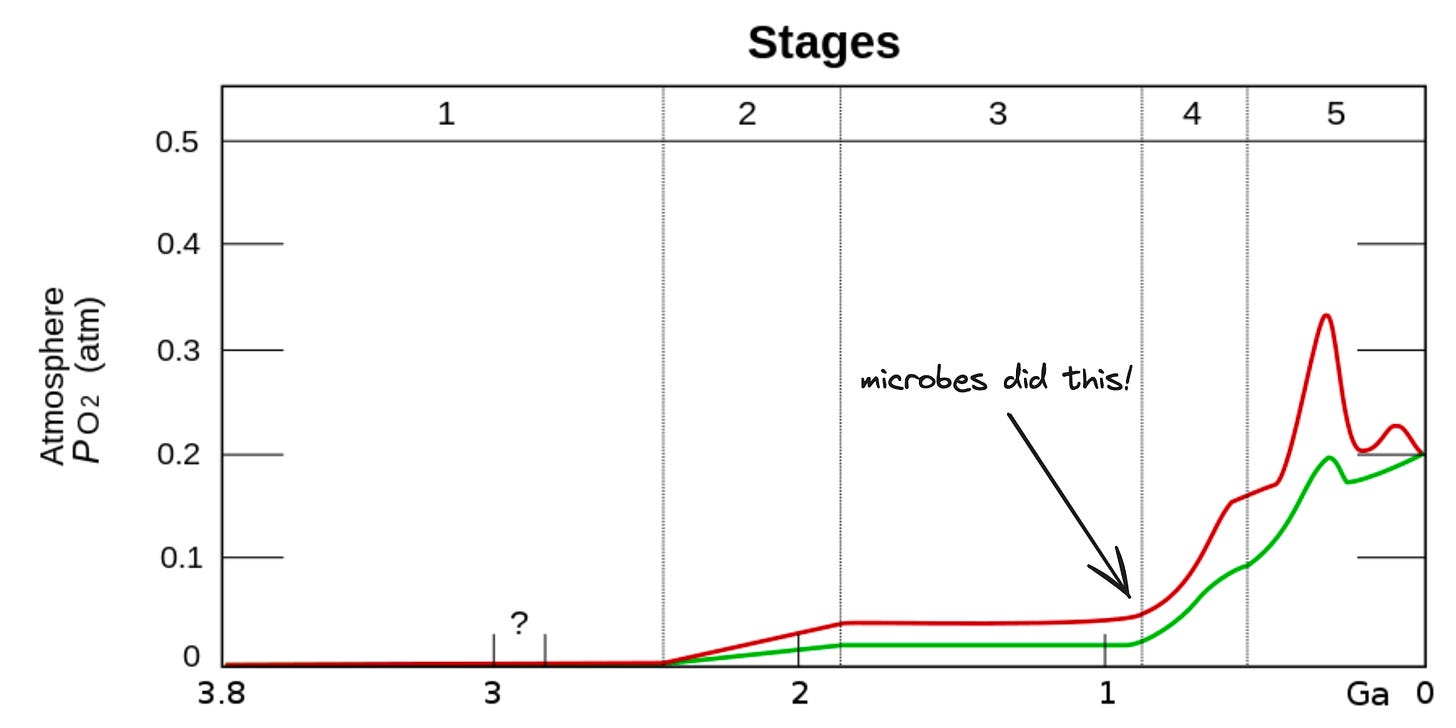
Still, in space, we don’t currently use biology for this job. On the International Space Station, CO2 gets captured from the air and pumped through a Sabatier reaction that outputs water and methane. The water gets used, and the methane gets vented out into space.
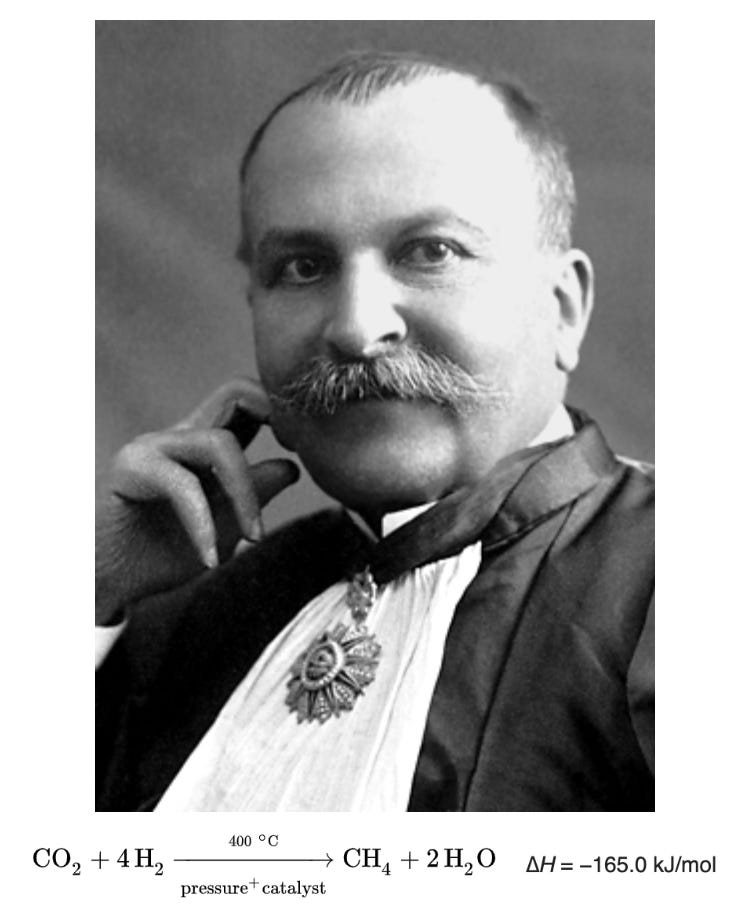
What if we used microbes instead? We could potentially cycle the CO2 we emit back into breathable oxygen. Even better, we could capture carbon and upcycle it, using it to biomanufacture food or materials like biologically produced concrete.1 This isn’t science fiction—researchers are already experimenting with use cases for cyanobacteria in a Martian context.
Beyond its potential role in carbon upcycling, biotech-based food production will be essential for more ambitious long-term space travel. The ecological design of microbe and plant-based bioregenerative life support systems is an active field of research.
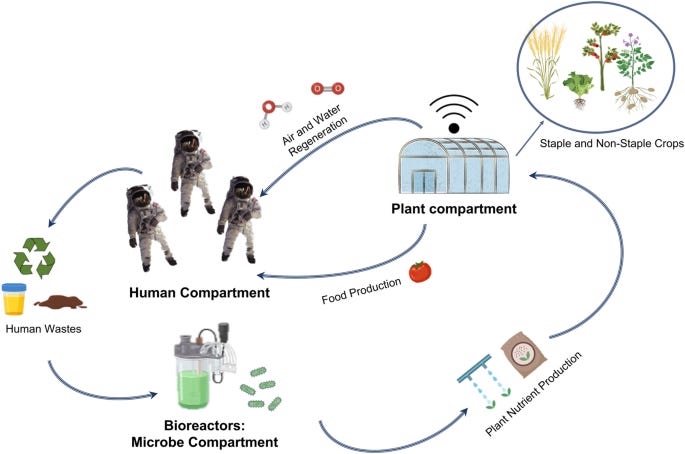
While alternative protein markets on Earth have grown more slowly than many anticipated, astronauts may be less picky. It’s highly unlikely that our current factory farming practices for animals will ever be viable in space, thankfully. Since we can’t take our ranches and cows with us, plant-based meat alternatives may be particularly compelling for longer missions. Soon, we could even bring bioreactors to produce cultured meat—which could dramatically improve astronaut diets.
Making medicines will be equally important. Many of the drugs we take with us from Earth will expire on longer missions. On Earth, synthetic biologists have spent decades engineering increasingly sophisticated metabolic pathways in microbes and yeast to produce drugs.2 And obviously, this is the best way to produce biological drugs already.
As far as space goes, the main challenge will be to automate the synthesis and downstream processing steps for bio-based drug production—and to shrink it into a form factor that is conducive to space travel. Essentially, we need to make a synthetic biology-powered compounding pharmacy in space.
It’s worth noting two direct applications of space medicine that are already being advanced to benefit Earth. First, space is a harsh physiological environment that accelerates aging, bone loss, and other types of disease. Research into astronaut health is likely to benefit human health on Earth. A company called Ursa Bio is looking to “solve health issues in astronauts during prolonged off-world living,” according to its founder, Yash Shevde. Second, there are distinct properties of microgravity that are advantageous for measuring and making active pharmaceutical ingredients. Varda is currently working to capitalize on this opportunity.
Most people consider food and drugs to be squarely within the purview of bioproduction. But what about materials—or even buildings? If we can’t take cows with us, we certainly can’t take concrete.
One potential solution to this problem is to leverage microbially induced calcite precipitation (MICP), which creates calcium carbonate (CaCO3). Calcium carbonate is a binding agent, meaning that MICP could be used to consolidate the space dust on the surface of Mars (called regolith) into concrete-like materials for construction.
But what if we take things a step further and grow our Martian habitats? NASA has researched and prototyped ideas for using the root-like fungal structures called mycelium to create self-constructing, self-regenerating outer space environments.
If we are clever enough, we could pack self-growing furniture and fixtures into these folded bags. Consider watching the concept video by Redhouse Architecture once, twice, or several hundred times to get inspired.
If you reexamine the initial flow diagram outlining all of these moving parts, it becomes clear that waste recycling is an essential node in the network. The ultimate vision is to have a compartment of microbial bioreactors that convert waste streams—from electronics and consumables to human excrement—into valuable inputs for food, medicine, and construction.
These types of “closed-loop” systems will be essential to minimize the need for Earth-based supply shipments, creating truly self-sustaining habitats for life on other planets.
What to take and when to make
If you can’t tell, many of these ideas are still concepts or rough prototypes—reducing space biomanufacturing to practice will require a consideration of techno-economics.
In a paper published in April, researchers from the Center for the Utilization of Biological Engineering in Space at Berkeley analyzed various mission scenarios to consider where biomanufacturing might have the greatest impact.
Not all space environments are the same. For Lunar missions, biomanufacturing likely has less immediate economic benefits. The moon’s proximity makes the logistics of resupply missions from Earth much more viable, and its harsh regolith and lack of atmosphere make in situ (local) resource utilization less appealing.
For Martian missions, conditions are the opposite. Mars has natural resources, including regolith with mineral oxides and iron oxides, an atmosphere with CO2 and nitrogen, and more than five million cubic kilometers of water-containing ice. This is a non-trivial amount—constituting nearly 20% of the size of the Antarctic Ice Sheet, which is one of the largest ice masses on Earth.
While it has more resources, Mars is also much harder to get to. A trip to Mars at the time of closest approach is more than 200 times longer than the equivalent trip to the Lunar surface. This makes the development of a closed-loop carbon cycle and infrastructure for in situ resource utilization even more important.
This is why the authors proposed gradual progression across four classes of mission:
Class 1 (Moon, stable logistics) — for shorter missions to the moon, like the first planned trips for Artemis, supplies can be brought along or resupplied from Earth. The focus should be on using this more stable mission class to test and de-risk bioreactor designs and push them up the technological readiness level (TRL) ladder by hardening them with miniaturization and automation.
Class 2 (Moon, disrupted logistics) — as we plan for more long-term infrastructure on the moon, all of the recycling infrastructure will be critical for success. Especially without many local resources on the moon, we will need to make the most of every molecule we bring with us.
Class 3 (Mars, rudimentary logistics) — when we turn our attention to Mars missions, all of the previous bio-infrastructure will need to be combined with solutions for manufacturing and in situ resource utilization.
Class 4 (Mars, developed logistics) — by the time we’re building permanent infrastructure on Mars, we’ll need to be able to build hardcore space biofoundries for local food, medicine, and material production.
In short, their qualitative techno-economic analysis showed that the farther outward we go, the greater the percentage of supplies and materials we’ll need to derive from biomanufacturing will be.
All in good time, my fellow space-obsessed bio-dreamers.
Future implications
What lessons or ideas can we draw from these plans for biotech-enabled sustainable space exploration?
First, this analysis shows that biotech isn’t synonymous with biopharma. While we currently equate biotech innovation with improved human health, it’s capable of delivering much more. Our terrestrial food and medical supply chains are already powered by biotechnology, but in the future, we’ll need to extend our reach into hard problems like recycling, energy, and manufacturing to ensure a flourishing and vibrant future on Earth. Biotech is essential for planetary health.
Second, space exploration may represent an under-explored go-to-market strategy for synthetic biology companies. One of the big challenges for synthetic biology to date has been achieving price parity with heavily scaled existing industrial practices. NASA could represent a customer that is more focused on the unique performance characteristics of biomanufacturing and is less price-sensitive.
Imagine starting out with a NASA partnership that provides a source of early revenue and potentially non-dilutive grant funding. If you can pull off building a Martian-grade automated compounding pharmacy, a bioreactor that can recycle electronic waste, or a self-growing Mycelium building, additional non-dilutive capital such as project financing could become available to scale your de-risked inventions into terrestrial markets.3
Just like CMOS image sensors and error-correcting GPS are NASA spinoff technologies, truly modular and local bioproduction and waste recycling solutions could also come from the space industry in the future.
In other words, if your life mission is to colonize space, maybe you should major in bioengineering instead of aerospace engineering.
Martian Myco-architect could become the sexiest job of the 22nd century.
But fundamentally, this is about life finding a way. Order popped into an otherwise chaotic universe, first in the form of cells. These cells found a way to coordinate in complex systems, producing everything from bumblebees, baboons, redwood forests, and dahlia flowers to neural networks capable of powering sentient minds—us. Life found a way to climb out of the ocean. Now, we need to find a way to place interstellar footholds to climb outwards again.
Life will find a way.
Thanks for reading this essay on biotech-enabled sustainable space exploration.
If you don’t want to miss upcoming essays, you should consider subscribing for free to have them delivered to your inbox:
Until next time! 🧬
A great current example is Antheia. One of their foundational papers is a remarkable feat of bioengineering.
If you’re interested in the prospect of using project financing for synthetic biology, stay tuned for an upcoming post that drills into this idea in more detail.
Great and (always) informative essay and so exciting to learn that many are already working hard on biotech solutions for space!
Thanks for writing this! It was nice to get an update on the research in this space; I'll definitely dig deeper and look into some of the references too.
A couple of years ago I took part in the NASA Deep Space Food Challenge in which my team tried to design a fully circular space-based food system, which taught me a lot about the challenges involved.
First off, plants are a no-go with current or near-future payload sizes due to their relatively low volumetric productivity; if you're going via the photosynthetic route you more or less have to use an optimised strain of a fast-growing cyanobacterium such as Synechococcus. It's not really possible to get a suitable macronutrient profile with wildtype, so you'd have to carry out significant metabolic engineering to make the relative production rates of the different macronutrient classes tunable and/or carry out secondary fermentation of the cyanobacterial biomass.
There are some challenges on the process side as well, namely sterilizing and degrading waste streams into substrates for the bacteria while minimising sludge formation, creating mixing and aeration systems for bioreactors that function in microgravity, and processing of biomass to remove and recycle excess nucleic acids, which are not safe to consume at the proportion they normally occur at in bacterial biomass. And that's not to mention processing all of this into something that would be edible. So it's a enormous challenge, but in an exciting way!
Unfortunately I'm not sure the institutional players are taking the right approach. The NASA challenge ended up having some pretty questionable winners (one of them violated mass conservation) which made the whole thing seem more like a publicity stunt than a serious competition. And the main EU project on closed-loop food systems, MELiSSA, is a mess IMO; it's trying to cram in too many different technologies and design approaches and not paying enough attention to the hard physical constraints involved.
Thanks for reading! :)