Welcome to The Century of Biology! This newsletter explores data, companies, and ideas from the frontier of biology. You can subscribe for free to have the next post delivered to your inbox:
Enjoy! 🧬
We are rapidly pursuing the industrialization of biotech. Large-scale automation now powers complex bio-foundries. Many synthetic biology companies are hellbent on scaling production volumes of new materials. A major concern is the shortage of bioreactors and fermentation capacity. While these all seem like obvious bottlenecks for the Bioeconomy, what if they aren’t? What if there is another way? Here, I’ll explore a different idea: the biologization of industry.
Few people have been more effective advocates of engineering biology than Drew Endy. As an early pioneer in the field of synthetic biology, he helped to launch the undergraduate majors in biological engineering at both MIT—which was the first of its kind in the country—and Stanford. His trainees have founded some of the leading companies in the discipline. Clearly, some of his ideas have taken root. However, the concept of the biologization of industry—which I view as one of the most compelling visions for the future of the Bioeconomy—seems to have remained dormant. Here is the core premise:
Biologization of Industry -- Many people default to a mindset of industrialization. But, why naively inherit a metaphor that dominated 19th century Britain? Biology is the ultimate distributed manufacturing platform. We are keen to explore and make true future biotechnologies that enable people to more directly and freely make whatever they need where-ever they are.
We exist on a beautiful planet that is completely carpeted in complex living systems. Some of these systems are microscopic in size yet are capable of blooming into populations that are visible from space.
Other organisms are insanely large, such as the Armillaria bulbosa fungus that spans at least 15 hectares and weighs more than 20,000 pounds. Biology manages to adapt and grow everywhere and is capable of both atomic precision and enormous scale. In other words, we inhabit a biosphere that is capable of producing more than enough to meet our needs. The holy grail of biotechnology is to learn enough of the language of Nature to put an end to disease and human scarcity. As Ginkgo CEO Jason Kelly puts it:

So… how do we get to a future where everything grows on trees?
At the outset, I’ll admit that this is an enormous question and I don’t have the answer. However, this is precisely the type of question that is worth spending time thinking about, and that can be a generative source of new ideas and directions. In that spirit, we’ll explore:
The industrialization of biotech
The Antibody Box
The Personal Biomaker
The Bionet
Let’s jump in! 🧬
The industrialization of biotech
The Industrial Revolution of the 18th and 19th centuries was a major inflection point for our species. Originating in Britain, this revolution laid many of the underpinnings of the modern world that we inhabit. The mechanization of work, the establishment of centralized factories, and the origins of modern capitalism can all be traced back to this tectonic shift. This all turned out to be a pretty big deal:
Gross domestic product (GDP) per capita began to skyrocket around the world after centuries of little change. We figured out how to make machines, and even machines that make machines. Early advances included the mechanization of textile production, but it’s hard to think of areas of materials production that haven’t since been industrialized. The industrialization of chemistry proved to be particularly consequential.
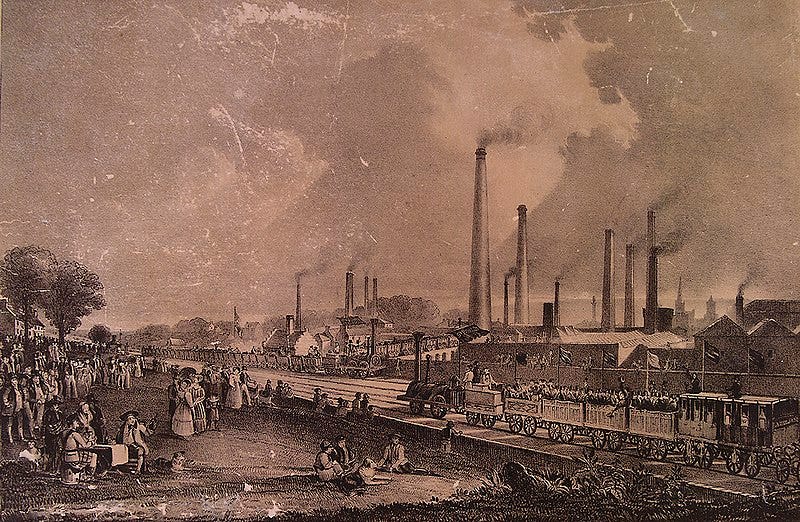
Efforts in scaling chemical production and expanding the diversity of molecules that could be produced ultimately led to the establishment of the petrochemical industry. Our world runs on these molecules. Our fuels and energy, clothing, materials and plastics, food (especially from fertilizers), and medicines are all products of modern industrial chemistry. Basically… Better Living Through Chemistry.
Despite the increase in production and abundance, industrialization has fundamental shortcomings and many negative externalities. Factories have a human toll. Many industrial methods are highly inefficient and produce enough pollutants to be a threat to planetary health. As we’ve seen during COVID and the Russian invasion of Ukraine, centralized manufacturing and complex global trade routes also introduce fragility into our world.
Current industrial production methods can’t be sustainably scaled.
The promise of biotechnology is that offers the solution to this problem. So far, we have mainly pursued the industrialization of biotechnology as a way to mitigate the consequences of existing approaches to production. The story of Genentech is an example of this. The first therapeutic produced using genetic engineering—insulin—already existed as a medicine. Before the use of genetic engineering, insulin was isolated and extracted from animals. Genentech’s major breakthrough was to replace highly wasteful and inefficient production methods.
The industrialization of recombinant DNA made it possible to harness cells as miniature factories for the production of drugs. Genentech scaled this powerful process in a centralized structure like a pharmaceutical company, and now operates as a subsidiary of Roche.
Early examples of consumer products produced using biotech have followed similar storylines. Pat Brown, who was a Stanford professor and inventor of the DNA microarray, founded Impossible Foods to create incredible meat alternatives using genetic engineering. Again, the goal was to mitigate the extreme consequences of industrial farming by producing an equivalent product with far less waste using bioengineering. The result is a new food company with centralized labs and factories that ships these meat alternatives around the world.
We are also starting to harness synthetic biology to tackle the issues of industrial chemistry. A Houston-based company called Solugen recently raised over $200 million in a Series D financing to continue growing the operations of their carbon negative molecule factory. This is an incredible example of what the industrialization of biotech can accomplish. Solugen has the potential to scale to profitably and abundantly produce commodity and specialty chemicals while removing CO2 from the atmosphere.
So far, the industrialization of biotech has produced powerful replacements for wasteful and inefficient methods of production. We can now generate insulin, and ironically, cheeseburgers, without sacrificing millions of animal lives. Companies like Solugen are demonstrating that we can use biology to build new chemical factories that don’t rely on digging fossils out of the ground.
Now, let’s turn our attention to what the biologization of industry might look like. As Endy likes to say, “biology teaches us that atoms are local.” This is a really important idea. Fundamentally, biological systems are built using only the local resources in their environment, and the information in their genomes. Put more simply:
The leaves on a tree don’t come from a factory and then get shipped to where the tree is going to be and taped and stapled to the twigs and branches. The photons and molecules arrive where the biology is going to grow and the biology grows locally.
How could we accomplish true local production? A starting point could be antibodies.
The Antibody Box
Antibodies are incredible molecular machines. They are large, Y-shaped proteins produced by immune cells to recognize and bind to specific molecules on the surface of pathogens in our bodies. When bound, they physically obstruct their target and serve as a signal for the rest of the immune system to respond to the pathogen.
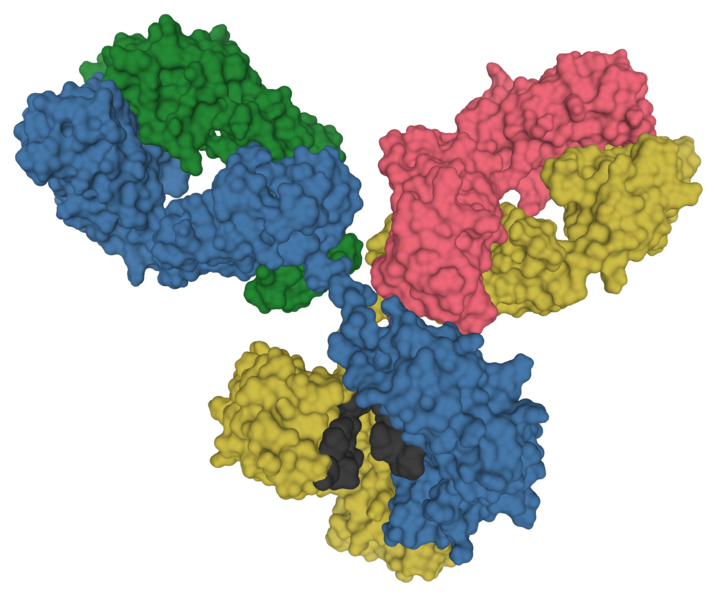
Due to their exquisite ability to recognize and bind to a wide variety of targets—which we call antigens—antibodies have become an absolutely central tool for molecular biology. Many of our most foundational techniques for quantifying proteins and detecting their location within cells rely on antibodies. We use antibodies to precipitate molecules out of solution, quantify proteins, visualize their location (IHC and IF) and do sensitive detection.
Antibodies are the workhorses of modern molecular biology. Due to their importance, there are a large number of commercial providers. Actually producing antibodies is a fairly involved process. Hybridoma technology is the most reliable method for producing large quantities of identical antibodies. It’s pretty crazy how it works:
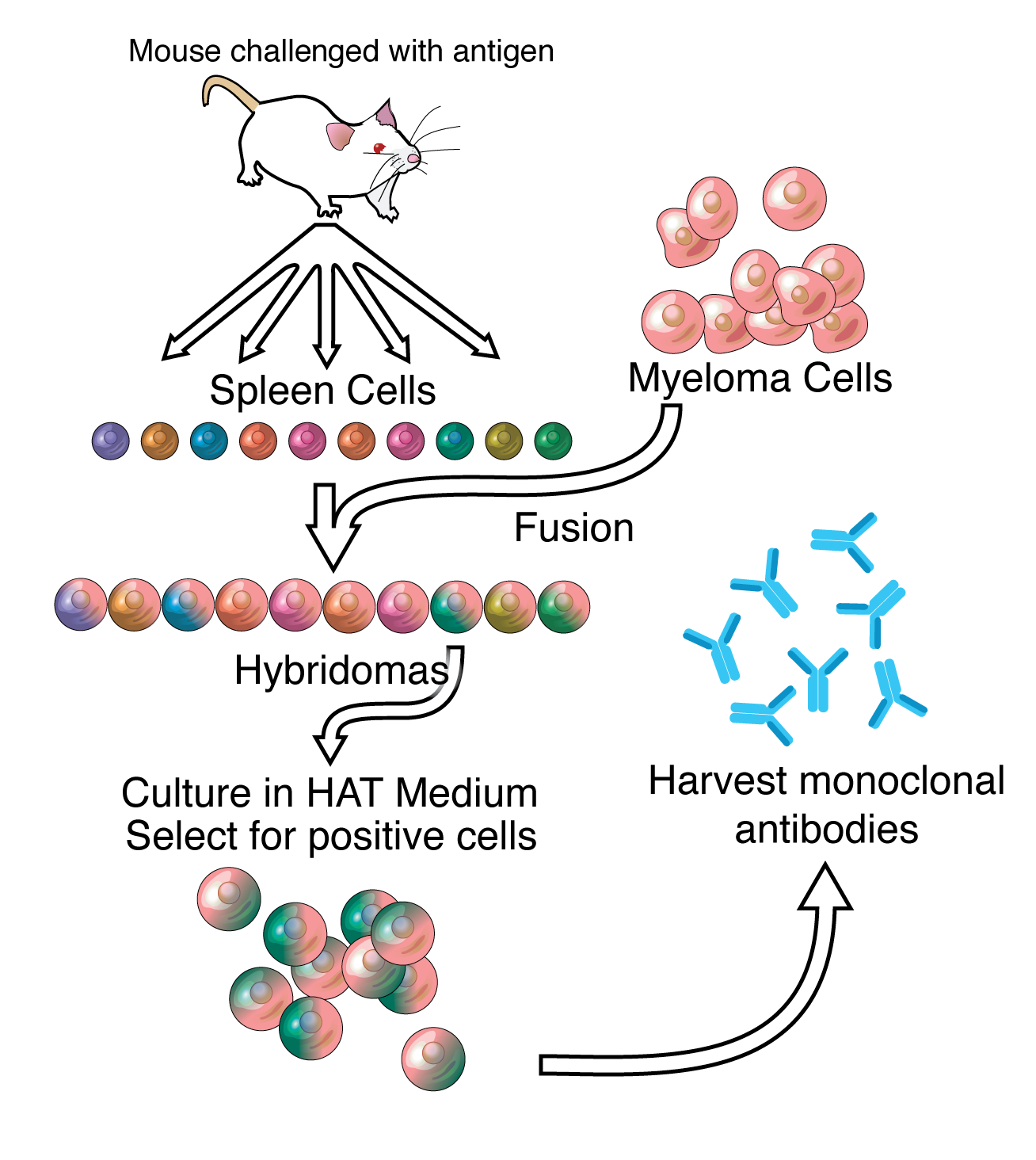
A specific antigen is introduced into a mammal with an immune system (typically a mouse). That animal’s B cells produce antibodies targeting the antigen. The antibody-producing B cells are then fused with cancer cells to create immortal cell lines that can produce abundant amounts of the antibody.
Similar to the story of Genentech learning how to produce insulin using bacteria as cellular factories, we have also developed approaches for the recombinant expression of antibodies. Antibodies are a more difficult application of this technology, because they are actually large structures comprised of four separate amino acid chains that are held together by bonds. To make production simpler, people have developed single-chain variable fragment antibodies, which make it possible to encode the binding regions of antibodies in a single amino acid sequence. This makes it easier to drop the sequence into bacteria for recombinant production.
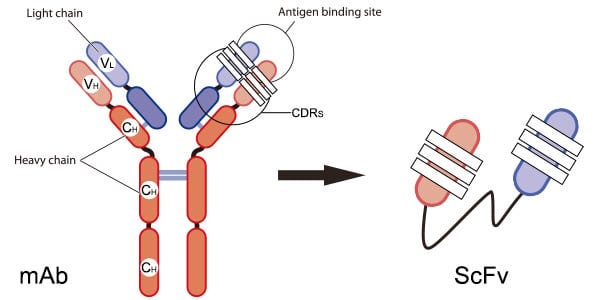
What if we could develop a technology to arbitrarily produce any antibody that we wanted, including entire four-chain monoclonal antibodies?
There are obviously an enormous number of engineering problems to solve before this is a reality, but let’s think about some of the building blocks at our disposal. An essential starting ingredient is DNA, especially segments long enough to encode every chain. A powerful tool here could be a DNA printer similar to what DNA Script is building:
Here, I’m not making a bet or judgment on a specific company. I’m arguing that with progress in enzymatic synthesis—and given the existence proof of the amount of DNA organisms all over the planet are constantly producing—it seems possible we can have reliable DNA printers in our lifetime.
Once you have a DNA sequence, work still needs to be done to express it. Thankfully, it’s likely that this process could soon be significantly easier. Companies like Nuclera are working to develop “desktop bioprinters” whose only input for optimized protein expression is DNA. Again, I’m not betting on any specific provider, I’m arguing that this form factor doesn’t violate any laws of physics or biology and that people are actively developing flexible bioprinters like this.1
So what happens if over time we combine all of this technology into a single box? It could be totally transformative. In the future, a researcher could walk up to the box and tap its screen. They could use an AI-assisted reagent selection platform like BenchSci to pick the best possible antibody for their target. With an antibody selected, they’d have an amino acid sequence:
…DSDGSFFLYSKLTVDKSRWQQGNVFSCSVMHEALHNHYTQKSLSLSPGK…
This would be compiled to DNA, synthesized, and then expressed. Any antibody they want could be generated. The move upwards in abstraction from raw material to the application layer—producing molecular tools—could potentially have important business consequences. To consider this, it’s first important to understand how molecular box companies make their money. The deep tech investor Ian Rountree posed the following question:

10X Genomics is a leading provider of infrastructure for single-cell and spatial biology. They sell powerful boxes that abstract away the complexities of these powerful measurement technologies. The answer to this question may be surprising. The overwhelming majority of 10X’s revenue (86% as of Q2 this year) comes from the sale of consumables. This is the primary mode of recurring value generation for this type of company—which requires a considerable markup in cost for scientists.
With a move to the application layer, what if this is no longer the case? An antibody box company could consider selling their instrument and consumables at cost (which would be very low) and instead generate their revenue by taking a percentage of all sales made and ultimately synthesized using their platform.2
This would be an early version of a biological app store model.
Just like Apple has their own set of native apps as well as an app store, this type of company could also have an internal design studio to produce new antibodies that they could sell and would earn the entirety of the proceeds for. This would resemble the model of Epic Games which sells the Unreal Engine to make new games, as well as their own games such as Fortnite.
Now, dear reader, humor me for one more leap in this chain of speculative reasoning. Outside of research use, antibodies are a valuable and powerful form of biologic therapy. They can be used to bind to disease targets instead of small molecules, and represent one of the largest growing classes of drugs. The production of biologics is intensely regulated and complicated. But what if, over time, we could chip away at the technical hurdles that prevent the rapid local production of specific antibodies personalized to individual patients? In other words, what if we could make a CGMP compliant antibody box?
During the COVID pandemic, we’ve seen an interesting example of the widespread adoptability of therapeutics made using recombinant protein technology. While the leading mRNA vaccines were expensive to produce and required a cold chain for distribution, scientists in Houston produced a recombinant vaccine now called Corbevax that could be rapidly made around the world. Developing the infrastructure for local antibody production could result in a much more resilient and accessible network of access to this rapidly expanding class of powerful biologics.3
The Personal Biomaker
Local antibody production would clearly be valuable for both research and medical applications. If we managed to solve the problems required to build this type of technology, how far away would we be from effectively having a Protein Box that wasn’t just limited to antibodies? What about a Protein Complex Box? If we continue down this trajectory, what would it take to make… a Personal Biomaker?
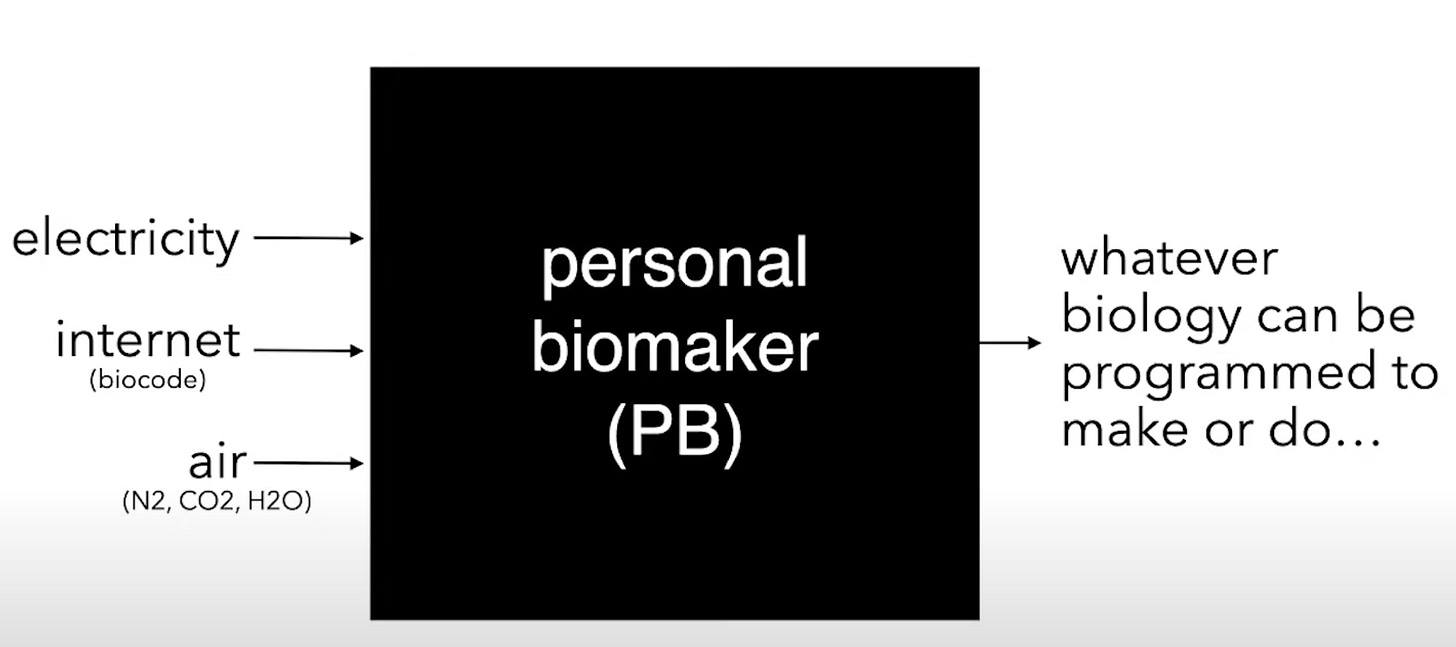
As Drew Endy recently asked, how could this box change the world? What if we had the capacity to make everything that biology can make, everywhere that biology makes it? Thinking back to the core concept of what the biologization of industry would unlock, we would be much closer to a future where we “enable people to more directly and freely make whatever they need where-ever they are.”
To reiterate, we know that enormous fungi are capable of spanning hundreds of acres of land with genetic continuity. Why would it not be possible—with sufficient knowledge—to instead grow a complex multi-room treehouse? We don’t know what the limit of our ability to engineer biology is, but we can be confident that we aren’t anywhere close.
Achieving this type of technology will likely require new paradigms for engineering biology. As Michael Levin at Tufts University argues, as bioengineers we are working with agential material: the cells and living systems that we work with already have their own idea of what they want to do. Effectively working with with these systems will require new formalisms and approaches in engineering and control theory. In the long run of this research direction, it’s possible to imagine something like an anatomical compiler:
A future system representing the long-term endgame of the science of morphogenesis, that reminds us how far away from true understanding we are. Someday, you will be able to sit in front of an anatomical compiler, specify the shape of the animal or plant that you want, and it will convert that shape specification to a set of stimuli that will have to be given to cells to build exactly that shape (no matter how weird - total control).
Clearly, developing an Antibody Box—let alone a Personal Biomaker or Anatomical Compiler—will require a substantial amount of scientific progress and engineering effort. My argument is that we should take this type of vision seriously and treat it as a priority. The future of the Bioeconomy doesn’t just have to be about industrial scale fermentation. Biology can make practically anything, practically anywhere. How do we learn to partner with that capacity more effectively?
The Bionet
The Internet is the crowning example of the type of distributed technological infrastructure that we are capable of building as a species. We have developed an interconnected global network of computers that makes it possible to send arbitrary information nearly anywhere in the world practically instantaneously. As Endy would frame it, the Internet has effectively made it possible to “disconnect information from a position in spacetime and move it around.” Bits are no longer local, but global.
This transition has really important implications for biology. We know that biological systems are built using only the local resources in their environment, and the information in their genomes. Coupling biotechnology with the Internet changes this. For an Antibody Box, researchers would be selecting from enormous libraries of possibilities, with the help of AI systems like BenchSci. This type of system would use the local resources in its environment in combination with a globally distributed set of designs.
The Internet + the Personal Biomaker = The Bionet.
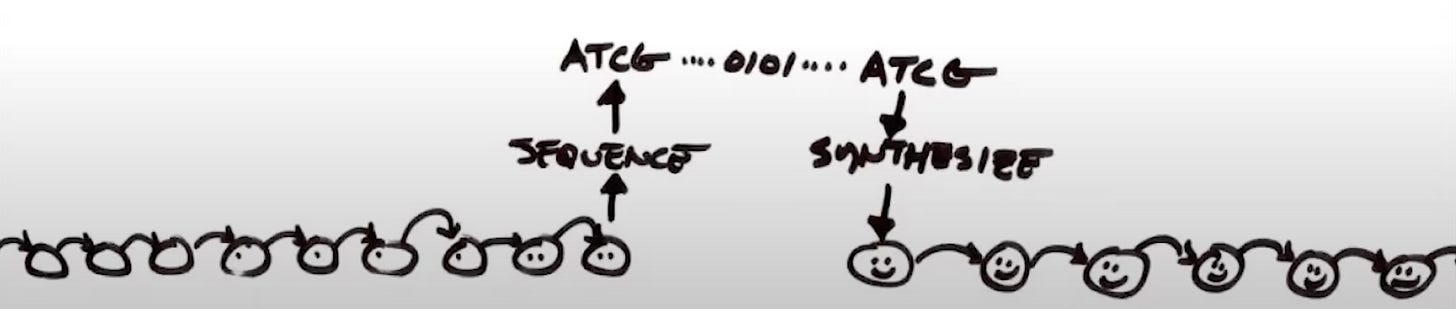
Alfredo Andere recently asked me a really important question on Twitter:

This question is a great framing of the value proposition of the Bionet: the marginal costs and distribution costs of actual material goods in the physical world could come to approximate the costs of distributing software products on the Internet. Let’s consider this…
Living in the industrialized world, we have giant retail stores that are packed with chemicals and detergents, medicines, and clothing and materials that have been shipped from all around the world in a complex supply chain. What if we didn’t need that? What if we could grow what we wanted locally? What if our supply chain was just biology?4
This is why the biologization of industry would look and feel very different from the industrialization of biotech. It would represent a radical shift for our global economy, and a major reorganization of the physical world. This distinction is not intended to represent a strict dichotomy. If we look at the structure of the Internet, centralized server farms have proven to be essential for providing cloud services and doing large-scale computation. The rise of edge computing shows how continuous the spectrum between centralized services and distributed computing can be. Similarly, powerful new bio-industrial factories like what Solugen and Ginkgo are building may serve as central nodes in the Bionet. But ultimately, one of the major lessons of biology is that planetary scale distributing manufacturing is possible. After all…
Atoms are local.
Thanks for reading this analysis of the difference between the industrialization of biotech and the biologization of industry. If you:
Disagree with this assessment and have useful feedback, or
Are building technology that supports the biologization of industry
Please consider reaching out. You can subscribe for free to make sure you don’t miss the next post:
Until next time! 🧬
This is likely to also be true for centralized industrial scale DNA synthesis. Twist Bioscience is aiming to target specific product verticals using their synthesis capabilities. One of their first major strategies is to do antibody discovery.
There has been some really exciting work on making vaccines more portable and eliminating the need for cold chain distribution. Other examples include progress towards on-demand biomanufacturing of vaccines using cell-free protein synthesis, and the development of a DNA-based vaccine platform by Alvea.
You may be thinking to yourself: being able to grow anything anywhere sounds kind of scary… how can we prevent this type of technology from causing immeasurable harm instead of good? I’m planning on surveying efforts in biosecurity in the near future.
I think this is a very interesting concept. I think the world is ceaselessly moving from decentralised to centralised and back again as we improve our technology and new capabilities open up.
For me, this has a personal element to it. I was diagnosed with Type 1 Diabetes back in October 2020. Now I am fortunate enough to live in Australia where access to Insulin is easy and affordable (almost entirely subsidised).
But what happens if there is a problem with supply of Insulin? Without it eventually I will die.
What if I could produce insulin myself by inserting an Insulin producing Plasmid into e-coil I’ve cultivated at home?
This isn’t beyond the realms of possibility. Using the kits provided here - https://www.the-odin.com/ I have already grown DH5A e-coli at home on LB Agar plates and introduced a DNA plasmid coding for a Green Flourescent Protein (GFP) which made them glow green under exposure to blue light.
With DNA coding for Insulin production inserted in the same way or via CRISPR Cas9 (currently doing this experiment but with different DNA payload) and a few processing steps off the back of it to isolate the insulin why can’t I produce my own like I can grow carrots in my back yard...?
You have exuberantly portrayed a captivating, albeit unconstrained vision, which positions itself as the top priority and the only way forward. The contrast between the industrialization of biotech and the biologization of the industry represents a dichotomy: humans as the primary engineers versus evolution as the primary engineer.
If we start from the First Industrial Revolution, driven by mechanization (the locomotion system), followed by electrification (blood vessels, peripheral nerve system), computation, and automation (brain and reflex movements), and the application of intelligent systems (AI), we can clearly observe humans employing forward engineering to shape the world around us, while aiming to mimic evolution.
On the contrary, the biologization of the industry signifies a paradigm shift in industrialization. In this case, we apply reverse engineering to comprehend evolution as the paramount engineering force, followed by divergent and re-engineering of the complexity of life, to usher in a brave new world.
Atoms are local. Priority is the ultimatum.