Welcome to The Century of Biology! This newsletter explores data, companies, and ideas from the frontier of biology. You can subscribe for free to have the next post delivered to your inbox:
Enjoy! 🧬
New scientific tools behave much like flashlights—they shed light on new parts of Nature that previously couldn’t be seen. Scientific progress is seemingly shaped by this. The development of new instruments is often followed by periods of heightened exploration. The pioneering molecular biologist Sydney Brenner described this phenomenon by saying “progress in science depends on new techniques, new discoveries, and new ideas, probably in that order.”
In the 21st century, our techniques for measuring and manipulating biology have dramatically expanded in power. Technologies for reading and writing DNA are both improving exponentially. Breakthroughs like CRISPR make it possible to edit genes in a programmable way. With this new foundational toolbox, biology is transforming into a quantitative and predictive discipline. This revolution is a central theme of this newsletter.
With this incredible new capacity, an important question emerges: what can we do now that wasn’t possible before?
Historically, it has been a serious challenge to begin work studying the genetics and molecular biology of a new organism. Because of this, researchers have organized themselves into model organism communities. Over the years, researchers have developed shared sets of molecular tools and resources for model organisms such as the bacterium E. coli and the fruit fly D. melanogaster. In his Nobel Prize lecture Nature’s Gift to Science, Sydney Brenner emphasized how central the toolbox for studying the C. elegans roundworm was for his work.
Modern technologies radically shift this equation. Our tools for rapidly reading, writing, and editing DNA are highly portable across the entire tree of life. We have a newfound capability to massively expand our exploration of entirely new organisms and biological phenotypes. We can renew our broad study of the natural world, this time doing so at the molecular level. Incredible traits like mammalian hibernation, the bizarre longevity of naked mole-rats, or the suspended animation of the African killifish can now be analyzed in genomic detail.
This renewed exploration of incredible organisms and phenotypes across the tree of life can be be broadly referred to as Extreme Biology.
Extreme Biology is one of the most promising research directions for making totally new discoveries. Low hanging fruit is likely to be far more abundant when we venture beyond the narrow set of organisms where most research currently takes place. Studying extreme phenotypes like cancer resistance, longevity, and hibernation may lead to biomedical insights that wouldn’t otherwise be possible. New molecular tools such as GFP, Taq polymerase, and even CRISPR—all discovered from organisms in Nature—may be found as we make the transition from “model organisms” to “organisms as technology.”
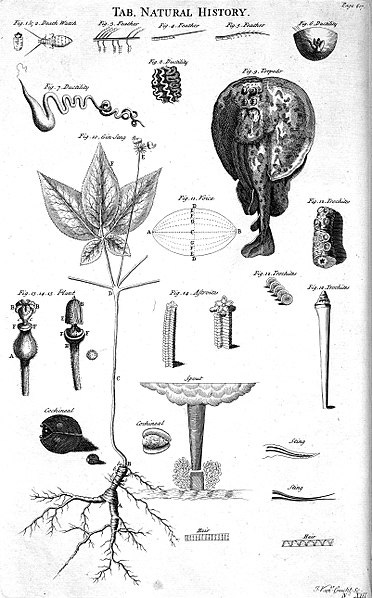
This essay is the culmination of a collaborative research process with Boom Capital and Fauna Bio. Cee Cee Schnugg, the founder of Boom Capital, came up with the term Extreme Biology after exploring and supporting this space as an investor. The team at Fauna Bio has been a pioneer in the application of comparative genomics to drug discovery. Together, we’ve attempted to synthesize some of the core concepts to understand about the world of Extreme Biology, what people are currently working on, and where things might go in the future.
Here’s where we’re going to go:
The model organism paradigm
The technology enabling Extreme Biology
Current exploration
Case study: Fauna Bio
Let’s jump in! 🧬
The model organism paradigm
It’s hard to overstate the importance of picking the right organism to study in the history of the life sciences. Organism selection is a multi-objective optimization problem. The organism must have the trait you care about in addition to being experimentally tractable. When Mendel was laying out the foundations of modern genetics, he needed an organism with a visually detectable heritable trait that could easily be grown and crossbred. For this, he chose peas.
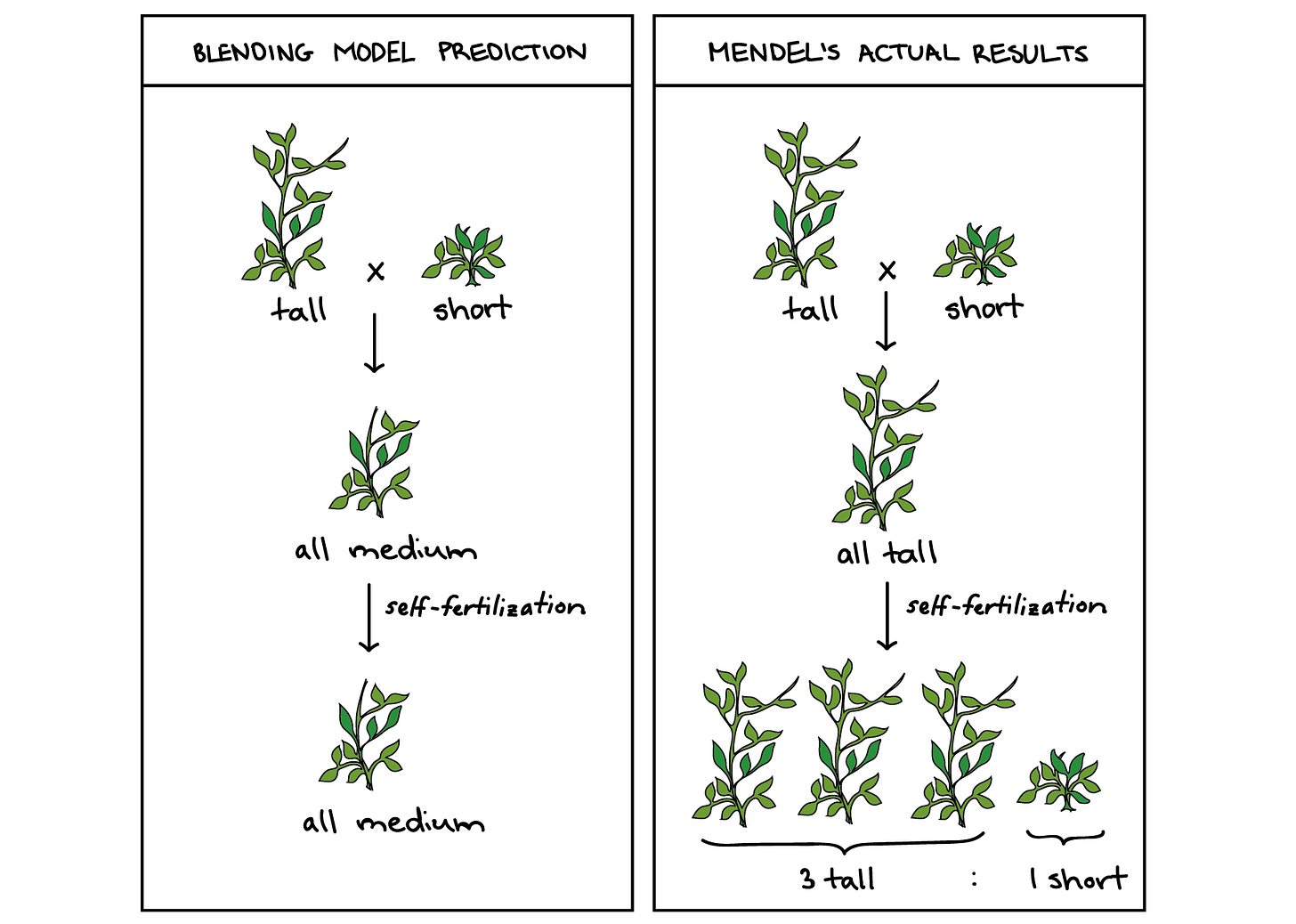
This turned out to be a really important choice. He had a simple system that he could use to iteratively test predictions about the basic rules of heredity as a single individual without complex instrumentation. While Mendel didn’t have a modern laboratory, he did have an abundance of time. Over many years, he painstakingly quantified the transmission of a variety of traits in his pea patch by hand. By making an excellent organism selection, he was able to independently launch the field of genetics.
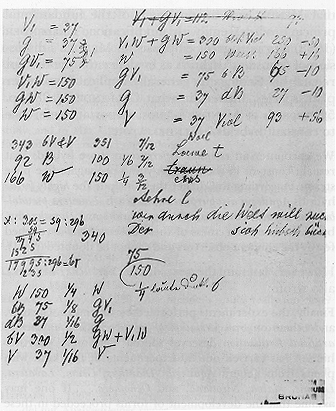
Fast forwarding along from the founding of the field, organism selection has remained a central part of genetics. With an understanding of the basic laws of Mendelian inheritance, a central question emerged: how do organisms actually maintain and transmit these heritable traits across generations? In tackling this problem, an unlikely hero emerged: the fruit fly Drosophila melanogaster.
In the early 20th century, a professor at Columbia University named Thomas Hunt Morgan began developing approaches to mutate and cross-breed flies. The fundamental goal was to be able to rapidly identify and trace heritable mutants across populations. Again, the selection of the fruit fly was far from a random choice. Flies could be rapidly bred, mutated, and crossed. Heritable traits could be quickly observed and counted with simple equipment.
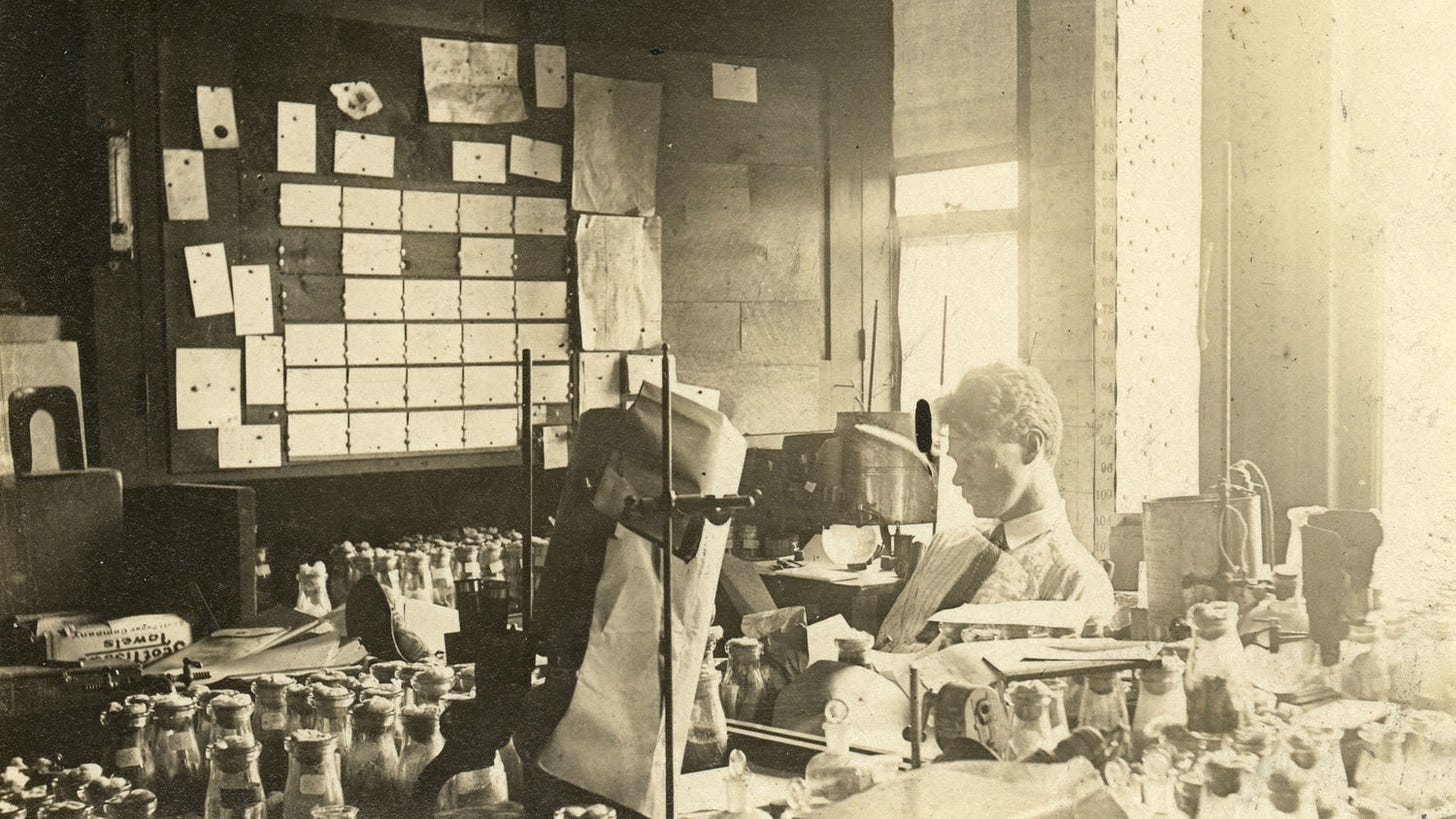
In the Fly Room at Columbia, Morgan and his team were able to use fruit flies to tease out crucial information about the physical basis of heredity. Through their experimental crosses and careful counting, they realized that the heritability of some traits—and therefore their underlying mutations—were linked to each other. This implied that there had to be some physical molecule both of the mutations were connected by. Decades before the double helix and nearly a century before the Human Genome Project, Morgan and his lab had discovered the chromosomal basis of heredity.
Because of the success of this experimental system, D. melanogaster became one of the most popular model organisms in the field of genetics. Scientists from around the world began crossing and breeding flies. Mutant strains were cataloged and shared. Resources, tools, and protocols were developed. The fruit fly model organism became the unifying tool for one of the largest and most productive research communities in experimental biology.
The profound path dependence based on this initial success can still be seen today: more than a century later the fruit fly is still one of the most widely studied model organisms. Not unlike the network effects that increase the utility of social media platforms as more users join them, model organism communities benefit as more scientists join them.
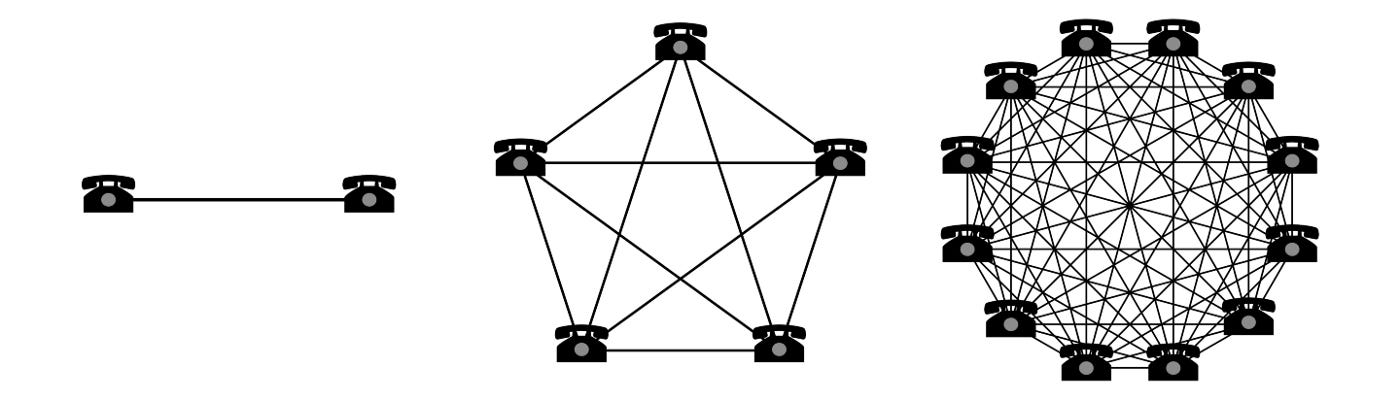
This is not just the case for D. melanogaster. As molecular biology—and later DNA sequencing and genomics—came online, the same network effects were present. The hard work of setting up new molecular tools was distributed across communities focused on specific model organisms. With the difficulty, time, and cost of DNA sequencing early on, any reference sequences—let alone reference genomes—became precious shared resources.
Now, the calculus for organism selection has been fundamentally altered. Again, organism selection is a multi-objective optimization problem. Historically, the traits being studied such as heredity and its physical basis permit selecting nearly any organism. Without sophisticated tools for measurement or perturbation, the obvious choice has been to select the simplest and most tractable organism. Peas, fruit flies, and worms have been ideal candidates. Is this still the case?
With modern DNA sequencing, if we want to study the genetics of a human trait, shouldn’t we start our research in humans? In the closing of Nature’s Gift to Science, Brenner says “C. elegans will continue to yield fruitful discoveries and insights in spite of my argument that we do not need model systems any longer for the study of human biology.”1
This sentiment can be seen in practice today. We are collecting massive population-scale datasets such as the UK Biobank and the All of Us initiative. These resources serve as the key fuel for modern human genetics. With new experimental systems like induced pluripotent stem cells, organoids, and humanized mice, the goal is to more accurately recapitulate human traits.2
Extreme Biology flips this focus on its head.
Instead of narrowing our focus onto a smaller set of human-like systems, what if we use the awesome power of our new technologies to radically expand our exploration outwards across the tree of life? What if we studied organisms specifically for their incredible differences from our biology instead of making them more like humans? What new molecular tools and insights into human health could we discover from this approach?
The technology enabling Extreme Biology
Alright, so let’s say that it does sound like a good idea to more broadly study the tree of life. Why is it actually more tractable to pursue this goal now? You may have already guessed, but the story begins with DNA sequencing. Again in Nature’s Gift to Science (I hope I’m convincing you to watch the lecture or read the transcript), Brenner observes,
The new technology had liberated genetics from the tyranny of the reproductive cycles of organisms and, in principle, could now be applied to any organism. Its power stems from the properties of DNA, which enable its isolation, amplification, and expression in simple microbes, and from the uniqueness of DNA sequencing, a technology that allows us to extract the essential information—the linear sequence of nucleotides—in DNA from any source: viruses, microbes, plants, and animals, as well as from molecules that can be copied into DNA.
Brenner made this insight into the universal power of DNA sequencing in 2002. In the time since, the technology has improved at a rate that even outstrips Moore’s Law in computer engineering:
This figure shows the Carlson curves named after the researcher Rob Carlson who made the observation that DNA-based technologies have been exponentially improving. It’s important to note that writing DNA—DNA synthesis—is also an incredibly important technology that is equally portable across the tree of life. It is an essential part of many measurement and perturbation technologies, as I’ve detailed before.
DNA sequencing alone has transformed our study of living systems. The new field of metagenomics is a powerful example. We can now sequence samples collected from the environment or the clinic and sequence the totality of the DNA that it contains—agnostic of the organism that it is coming from. After sequencing, it is possible to resolve which species—and even entire genomes—are present. Metagenomics has reshaped the study of the evolution and function of microbes and viruses.
But the story doesn’t just end with DNA sequencing and synthesis. An essay from Arcadia Science captures this beautifully, saying “we are at an incredible moment in scientific history when an array of distinct but complementary technologies for accessing and exploring diverse species are rapidly maturing, including genome sequencing, gene editing, microscopy, mass spectrometry, phylogenomics, machine learning, and many, many more.”
Our powerful sequencers, microscopes, spectrometers, and computers are agnostic to which organism they are used to analyze. We have never before been better equipped to expand our studies across the natural world. Sequencing, supercomputers and improved alignment algorithms can now paint global pictures of evolutionary relationships between organisms. Our gene editing technologies and tools from biochemistry and imaging can provide the resolution necessary to zoom into new parts of the evolutionary tree where we want to take a closer look.
Current exploration
Where is Extreme Biology right now? Some academic fields have prioritized the establishment of new models that may better answer their most fundamental questions. One notable example is the number of new systems in the field of aging research. Labs have worked together to establish tools for organisms with extreme age-related phenotypes such as the naked mole-rat which exhibits cancer resistance and exceptional longevity for a rodent. On the other end of the spectrum, the African killifish is the vertebrate with the shortest lifespan that can be bred in captivity, making it an ideal choice for rapid functional studies of aging.
While academic efforts to explore extreme organisms and phenotypes do exist, there are institutional barriers to more rapid expansion. Even with new technologies, Extreme Biology has a higher upfront cost than continuing existing work on established models. It can be challenging to fund this type of work within a system that primarily assesses prior work from individual labs when deciding how to allocate resources.
Focused Research Organizations (FROs) are one approach to addressing this type of funding gap. The core idea of the FRO is to remove bottlenecks to rapid progress that are are a result of our current approaches to funding and organizing scientific research.3 Cultivarium, which was one of the first FROs to be launched, is dedicated to Extreme Biology. Their mission is to help “access the genetic potential of the biosphere.” To do this, they focus on developing open-source tools and technologies that make it easier to culture and engineer novel microbes—an important strategy given that the microbial world has been one of the most abundant sources of new molecular tools.
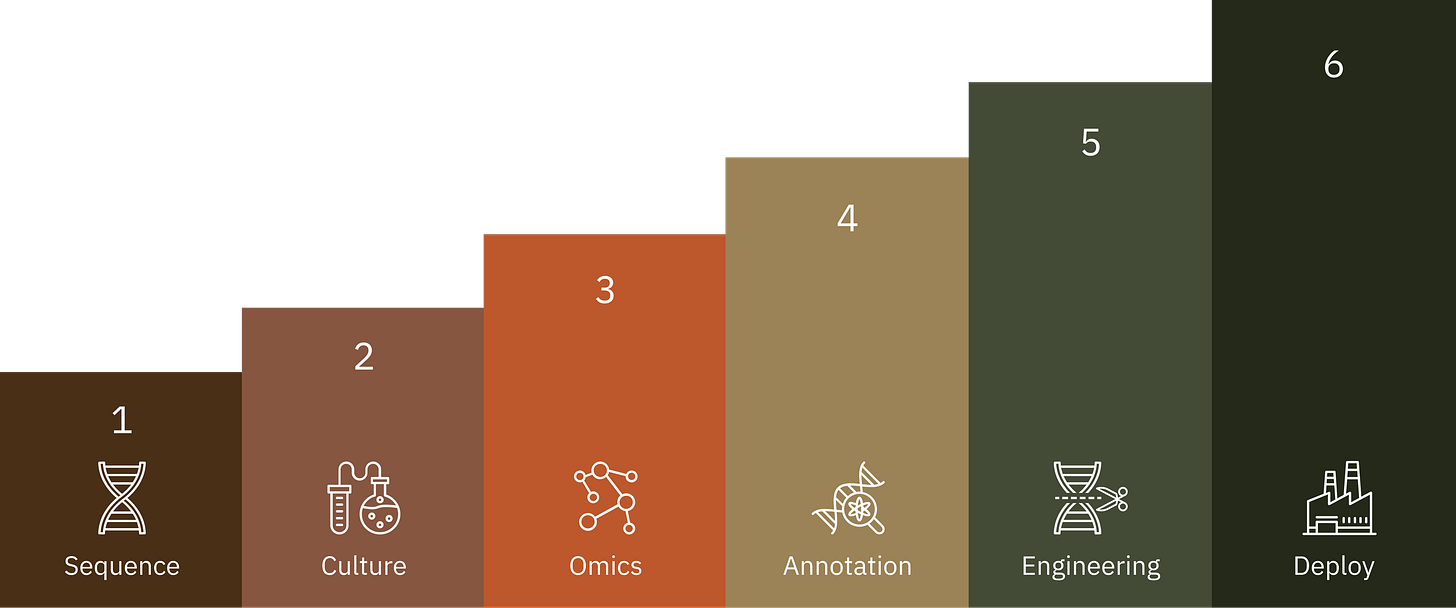
The tools produced by Cultivarium may further reduce the activation energy required for labs to pick new organisms to study or engineer. However, there is another factor that is accelerating Extreme Biology—there is immediate translational potential in the exploration of novel organisms and phenotypes.
There are several clear commercial opportunities for Extreme Biology.
To start, there are several tangible use cases within the market that is the 800-pound gorilla of biology: therapeutics. Extreme Biology is poised to accelerate the discovery of new drug targets, and new molecules and modalities.
From a target perspective, there is considerable evidence that drug targets with genetic evidence of disease association are twice as likely to be approved. By casting a broader net with comparative and functional genomics, Fauna Bio is aiming to discover a larger number of highly specific targets with genetic evidence (more on Fauna shortly). Another startup called Variant Bio is working to leverage targeted sequencing of human outlier traits based on a similar rationale.
There is a considerable amount of commercial activity in the molecules and modalities space for Extreme Biology. There is a strong historical precedent for this strategy—this is how many of our best medicines have been discovered. It was estimated that between 1983-1994, 39% of new approved drugs were natural products or derived from natural products. This number was even higher for antibacterial and anticancer drugs, of which 60-80% were natural products.
Some of the biggest blockbuster drugs were originally discovered in plants, microbes, and animals. Statins, which generate nearly $25 billion of annual sales in the U.S. alone, were originally discovered in fungi.4 Animals have also been a rich source of potent drugs, with ACE inhibitors having been discovered in snake venom, and GLP-1 agonists coming from lizard venom. It is evident that the chemical space synthesized by living organisms is vast and enriched for bioactivity. Despite this, the pharmaceutical industry began to shift away from the use of natural products starting in the 1990s towards rational design—where molecules are chemically designed based on knowledge of a target.
There were several factors associated with this shift. There were a number of challenges in sourcing, isolating, and de-replicating new natural products.5 Now, a number of promising companies are harnessing the new tools of Extreme Biology to accelerate a shift back to the exploration of Nature’s rich chemical space.
Aiming to tap back into a powerful source of potent medicines, Enveda Biosciences is building a platform combining metabolomics and AI to accelerate the discovery of new medicines from plants. Hexagon Bio is also aiming to “turn nature’s DNA into new medicines” but is searching through microbial genome space for small molecule inhibitors.
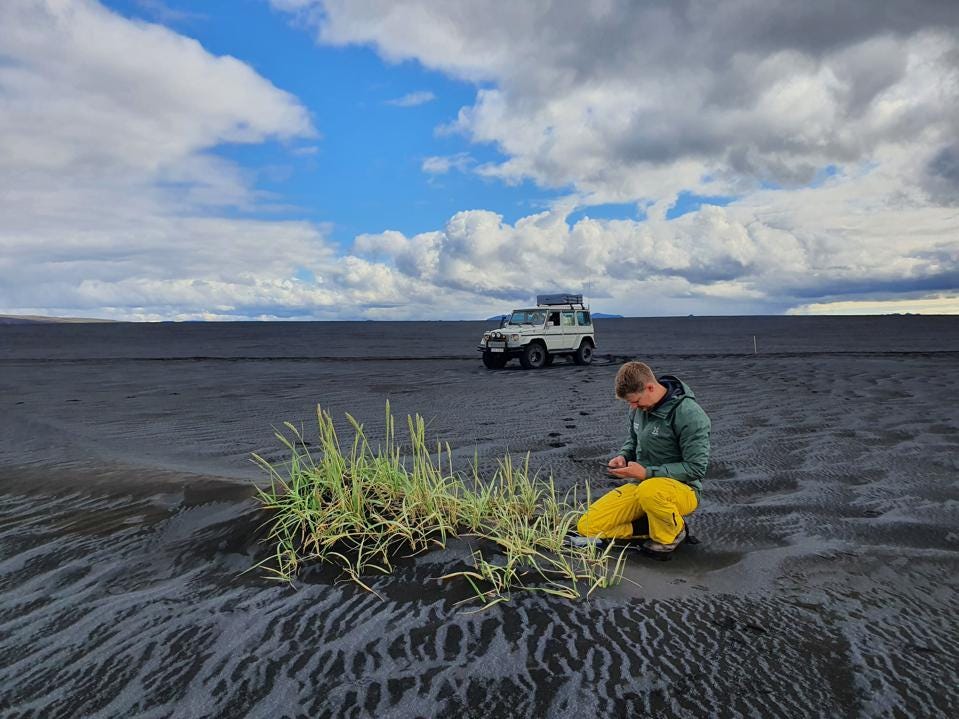
Given the rich history of discovery in microbes, there are several companies similarly focused on metagenomic discovery. Basecamp Research is building a microbial discovery platform for new protein products, and just announced their Series A financing this week. Wild Biotech is working to establish a massive database of the microbiomes of wild animals. Metagenomi has established several notable partnerships—including with Moderna—to expand their search for novel nucleases for gene editing within microbial genomes.
Another company turning to Nature for inspiration is Peel Therapeutics. Peel aims to translate evolutionary solutions into medicines. One of the programs in their pipeline is based on my favorite example of Extreme Biology—cancer resistance in elephants based on p53.
Together, all of these companies are tapping into the incredible power of the natural world to accelerate therapeutic discovery. A key theme is that these companies are using powerful new technologies to eliminate bottlenecks to natural product discovery that made the transition to rational design seem worthwhile.
The sheer size of the therapeutics market produces a strong gravity well for biological product development. Any biotech entrepreneur can hear sirens singing songs of blockbuster drugs in the distance. Despite this, biology is the ideal technology for many of our most pressing planetary problems and there are many enticing non-therapeutic markets that are hungry for new products and solutions.
A major open question in the 21st century is how to adapt to climate change while feeding a growing population. Extreme Biology is poised to play an important role in strengthening our food supply chain and making it more sustainable. Several companies are directly focused on using solutions from Nature to improve farming production. The UK-based startup Wild Bio is aiming to explore the genetics of wild plants for new ways to enhance crop yields and promote gigaton-scale carbon mitigation strategies. A recent IndieBio company called PunaBio is developing a new seed treatment based on extremophile microbes to expand the range of environments where crops can be grown while increasing their yields.
Consumer facing bioproducts are a growing segment of the Bioeconomy—and are another area where a broader exploration of novel organisms will play an important role. The growing plant-based foods market will benefit from startups like Prose Foods (an exciting Boom Capital portfolio company) that are accelerating the search for new plant proteins to improve the consumer experience, health benefits, and sustainability of this product category.
Nature’s design space expands far beyond food products. There are tangible opportunities in large established markets like the $500B beauty and skincare industry, where Seaspire is repurposing chromatophores to make better and safer sunscreens and skin products. Startups like Checkerspot are also aiming to make totally new types of biomaterials by engineering microalgae and other microbes. If history is any indication, new materials can lead to entirely new products and markets that don’t yet exist.
The set of companies highlighted here is by no means an exhaustive map of the growing space of commercial Extreme Biology efforts. However, noting specific examples helps to reveal the fact that the general strategy of repurposing novel natural solutions from across the tree of life extends beyond any single market.
Case study: Fauna Bio
Now, we’ll zoom in on Fauna Bio to more closely analyze what an Extreme Biology company looks like. Fauna is building a platform to leverage extraordinary genomics for human health.
To understand this vision, it’s important to understand the company’s origins. The first kernel of the idea was formed for Ashley Zehnder—now CEO of Fauna—when she was making the unusual transition from veterinarian to cancer biologist. Having entered veterinary school at age 20, Ashley was on the fast track to achieve her childhood dream of practicing animal medicine.
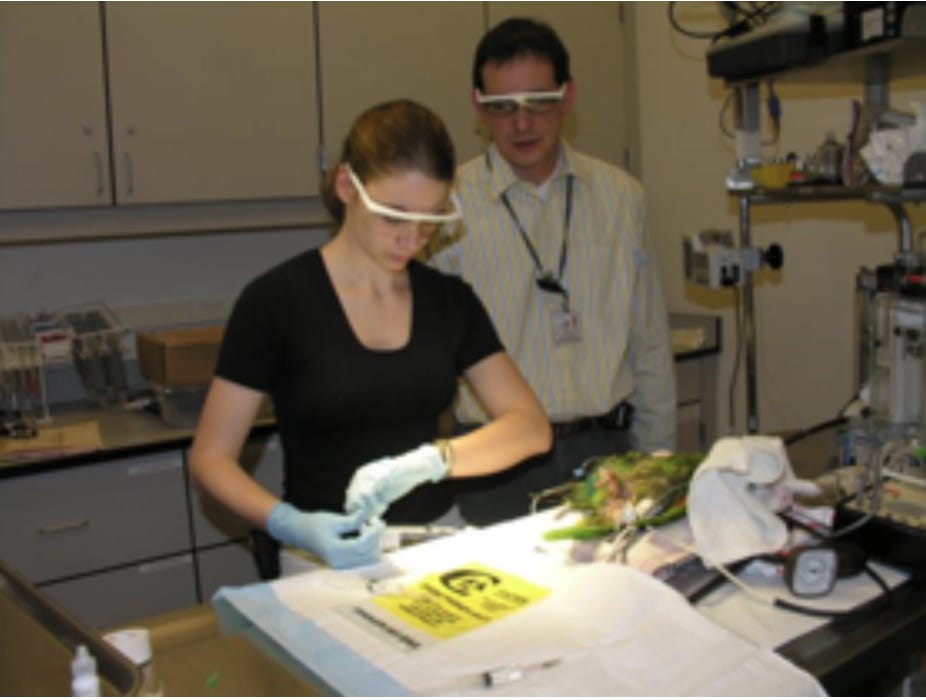
In the 2008 market crash, veterinary medicine was hit particularly hard. Ashley’s odds of landing an academic veterinary job effectively went to zero. Unwilling to give up science for a life entirely in the clinic, she doubled down and started a PhD in cancer biology at Stanford. Even during her interview process, it became abundantly clear that her experience as a vet lended her a unique angle on the study of cancer.
Despite the historical importance of studying cancer in animals—Rous sarcoma virus, the first cancer-causing virus to have been described, was identified in chickens—she realized that there was effectively no work being done to understand why cancers behaved so differently in different animals. As Ashley says, “a melanoma in a dog doesn't behave the same as it does in a corn snake or on the toe of a rabbit. Also, there were clear differences in physiology. Birds tolerate much higher doses of radiation than rabbits or guinea pigs, but why?”
In her time as a postdoctoral fellow with Carlos Bustamante at Stanford, Ashley overlapped with kindred spirits. Her colleague Linda Goodman—now CTO of Fauna—had taken her own winding path to focusing on mammalian biology beyond humans. Inherently quantitative, Linda gravitated towards population genetics and computational biology where the tools of math and statistics could be used to reveal biological and evolutionary patterns.
During her graduate work, Linda did careful genetic research to uncover genetic deletions in the human genome that were associated with lower cholesterol. Despite the genetic novelty of her findings, there was a wrinkle—the effect sizes on cholesterol levels were so small that it couldn’t serve as a drug target. Frustrated by this, Linda began to look for opportunities to use “all of evolution—not just human differences—to inform disease biology.” By looking more broadly across the evolutionary tree, it would be possible to find variants and biological adaptations with much larger effect sizes. This idea led her to the Broad Institute where she was a member of the 200 Mammals Project, and then to Stanford where she met Ashley.
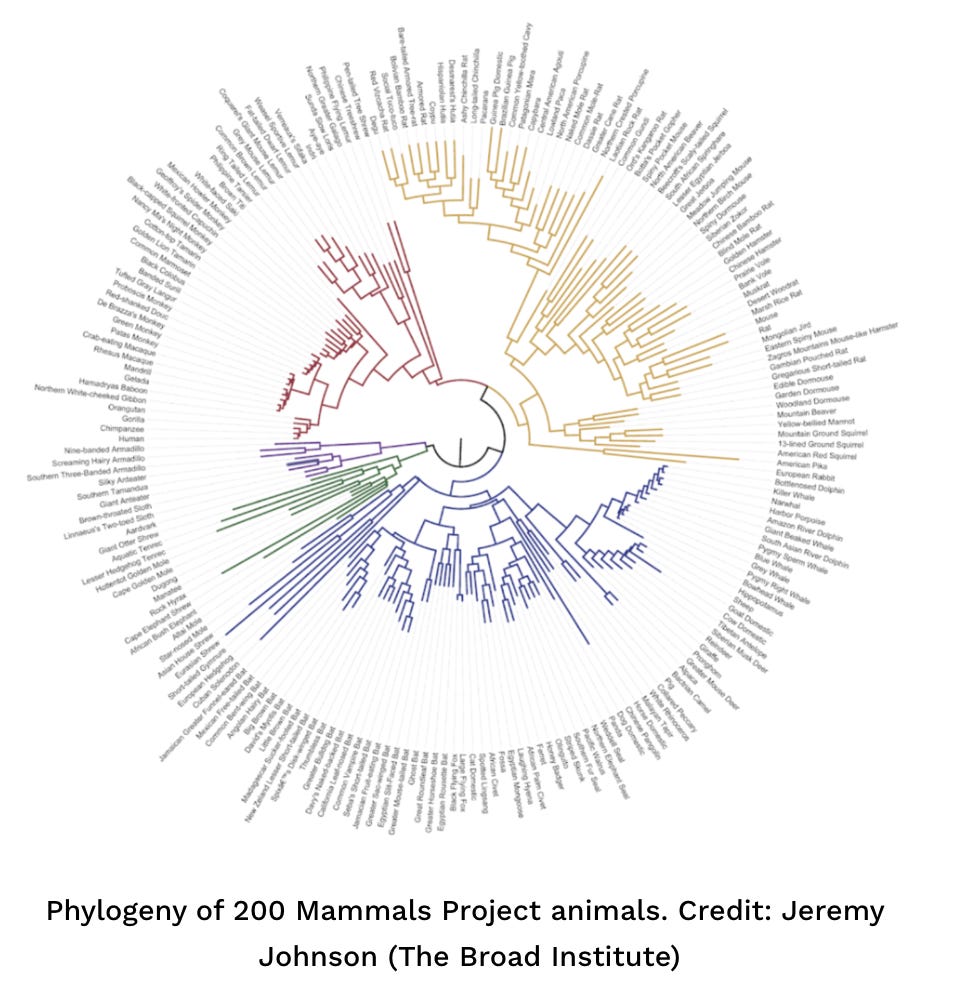
Between Ashley’s vision for exploring extraordinary animal phenotypes to understand human disease and Linda’s deep technical expertise in comparative mammalian genomics, the foundation for Fauna Bio was nearly in place. There was still an open question: what extraordinary genome should they start with?
Here, data from their fellow postdoc Katie Grabek—now CSO of Fauna—made the answer abundantly clear. Katie had spent her PhD and postdoc using the tools of modern genomics to explore a truly extraordinary trait—hibernation. How is it possible for tiny 13-lined ground squirrels to survive long periods with body temperatures so low that it would kill humans?
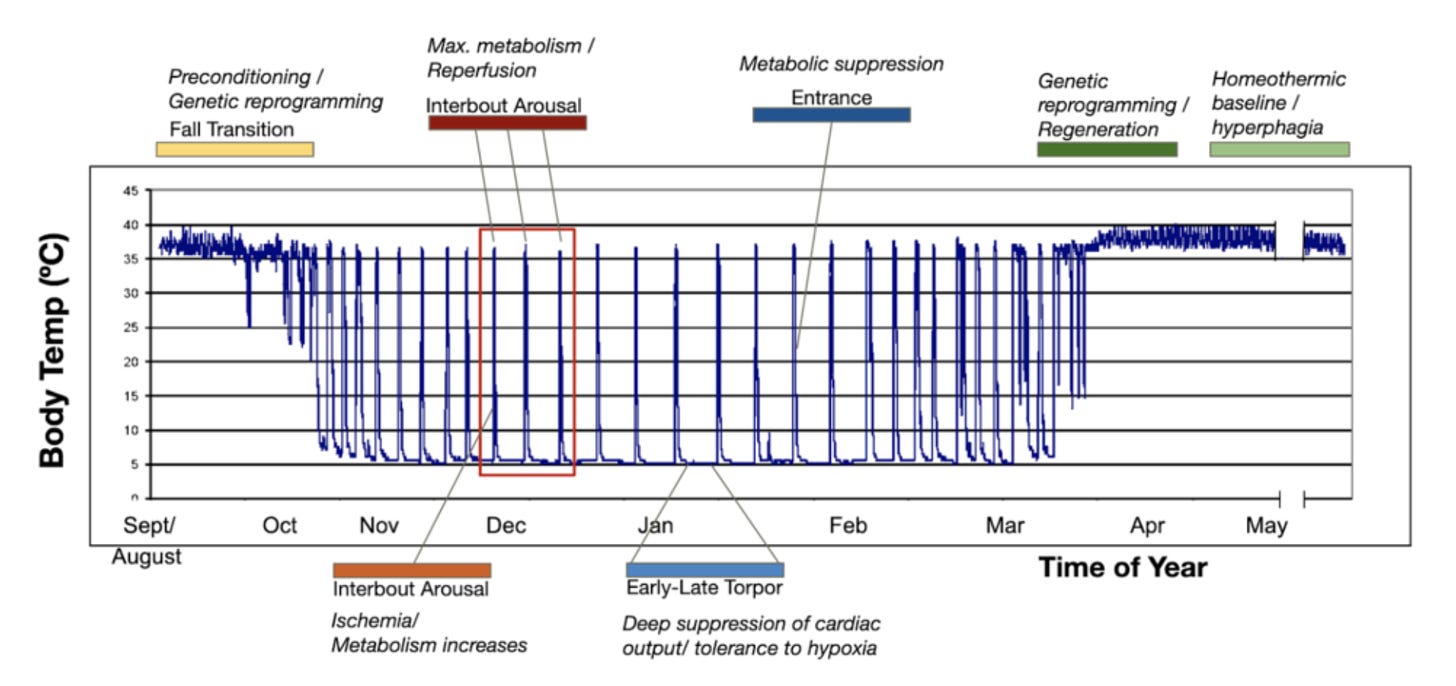
It turns out that some of the adaptations that made hibernation possible also made heart disease impossible. In order to endure such major fluctuations in body temperature and blood flow, hibernating mammals have adapted to conditions that would cause heart attacks and strokes in humans. Each genetic detail about hibernation that Katie discovered had translation implications for a massive disease category.
When Ashley, Linda, and Katie joined forces, Fauna Bio was born. The broad vision of the company is to use data from extraordinary animals to improve human health. The starting data platform is anchored around the translational potential of hibernation biology. Since launching, Fauna has constructed the most in-depth biobank of hibernation measurements in the world, collecting over 22 distinct tissue types across 13 time points. The result is a database comprised of transcriptomes, proteomes, and epigenomes—totaling over 22B sequencing reads—a feat previously unimaginable for a new startup before the Carlson curves kicked in.
All of this data is fed into a computational platform that searches for functional gene networks that are responsible for preventing disease. These gene networks are the basis for a broad comparative analysis across over thirty species—including humans—to pinpoint how they have evolved. The gene expression signatures underlying extreme adaption can be used as a platform for drug repurposing, where the goal is to identify existing molecules that produce the adaptation signature. While compound prediction is a starting point, the platform is ultimately modality agnostic—serving as a source of high-quality genetic targets that can be drugged with either small molecules or biologics.
Using their extraordinary genomics platform, Fauna has already identified several promising pre-clinical compounds—targeting diseases of the heart and lungs as well as the brain—and has established several partnerships with leading academic and pharmaceutical institutions.
Fauna Bio is an example of a promising translational strategy for Extreme Biology. By leveraging the power of modern genomic technologies, they have developed a world-class data platform for a novel and understudied organism with a unique phenotype of heart disease resistance. This data is the fuel for a computational engine that combines evolutionary analysis with machine learning to identify new drug targets with genetic evidence.
Ultimately, these initial steps represent a small sliver of Fauna’s vision. The goal isn’t to stay focused on ground squirrels or hibernation phenotypes indefinitely. The foundation that Fauna is building is based on the power of genomics and evolutionary analysis. Their search across the space of extraordinary organisms for new and highly specific drug targets has only just begun.
Final thoughts
Throughout the history of the life sciences, it has been a serious challenge to begin research of a novel organism—especially at the cellular and molecular level. For this reason, biologists have organized in model organism communities to make progress. Now, in the postgenomic era, we have an incredible new opportunity to read, write, and edit DNA across the tree of life. The space of possible exploration has rapidly expanded. The scientific and commercial opportunities are enormous.
We are seeing the birth of a new generation of therapeutics companies with an emphasis on finding the solutions that Nature has already invented—across plants, microbes, and animals—by harnessing the tools of genomics, synthetic biology, and computation. This logic extends across the rest of the growing Bioeconomy, where labs and companies are leveraging novel organisms to move beyond developing drop-in replacements for existing products. The goal is to cultivate evolutionary technologies that extend beyond what we can currently engineer.
Across The Century of Biology, Boom Capital, and Fauna Bio, we are extremely excited about what the future holds for Extreme Biology. If you are a scientist or entrepreneur working in this space, we’d love to hear from you. We are always looking to learn about more labs and companies doing foundational work in this space.
We are at the very beginning of our genomic exploration of the natural world. While many groups are now venturing beyond traditional organisms, our sampling of the biosphere is still incredibly sparse. Extreme Biology aims to accelerate this discovery process—with the goal of discovering the breakthrough medicines and products that Nature has already invented.
Thanks for reading this essay about Extreme Biology. This was the output of a collaborative research process with Cee Cee Schnugg at Boom Capital, Michael Snyder who helped with research on this theme early on, and several conversations with Ashley Zehnder and the Fauna Bio team. As always, a massive thank you to my editor Kelda.
You can subscribe for free to make sure you don’t miss the next post:
Until next time! 🧬
He followed this by saying “However, there are many aspects of humanity that we still need to understand for which there are no useful models. Perhaps we should pretend that morality is known only to the gods and that if we treat humans as model organisms for the gods, then in studying ourselves we may come to understand the gods as well.”
This was a big part of my early scientific training. At the Cancer Vaccine Institute, we spent a lot of time establishing better mouse models for pre-clinical testing of the vaccines we were developing.
If you want to learn more about FROs and some of the new applied metascience experiments in the life sciences, you should check out the deep dive that I wrote with
on this topic.The story of Dr. Endo, Merck, and the discovery of statins is worth reading.
IP protection around natural products has also been said to have increased in difficulty, but the landscape of patent law is always evolving.
> When Mendel was laying out the foundations of modern genetics, he needed an organism with a visually detectable heritable trait that could easily be grown and crossbred. For this, he chose peas... By making an excellent organism selection, he was able to independently launch the field of genetics.
One interesting part of Mendel's story, which furthers your point: his results didn't replicate in other organisms! He wrote to other botanists, who said he needed to replicate his results in other plants. When he tried to replicate the results in hawkweed, testing in excess of 5000 florets, he got completely contradictory evidence... because it turns out that most hawkweed seeds are produced asexually.
I think I first read about this in Mukherjee's _The Gene_, but refreshed my memory with [Seeds of doubt: Mendel’s choice of _Hieracium_ to study inheritance, a case of right plant, wrong trait](https://link.springer.com/article/10.1007/s00122-016-2788-x) while writing this comment.
Thanks, this was a really interesting and informative piece. Looking forward to more articles on this topic.